The Mysterious Case of the Renegade Receptor
by Neil Caudle
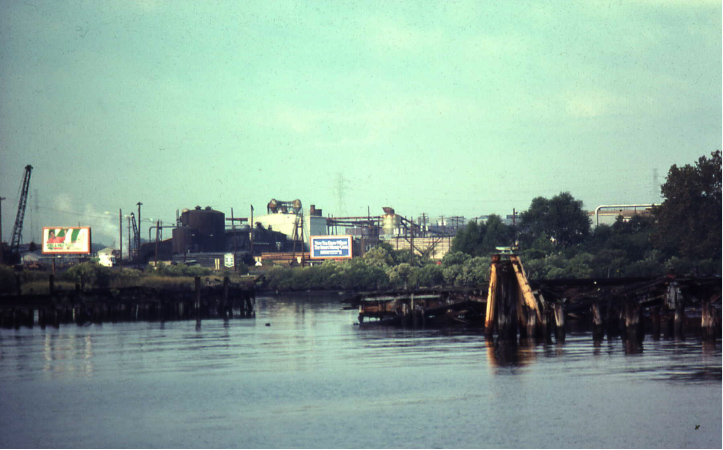
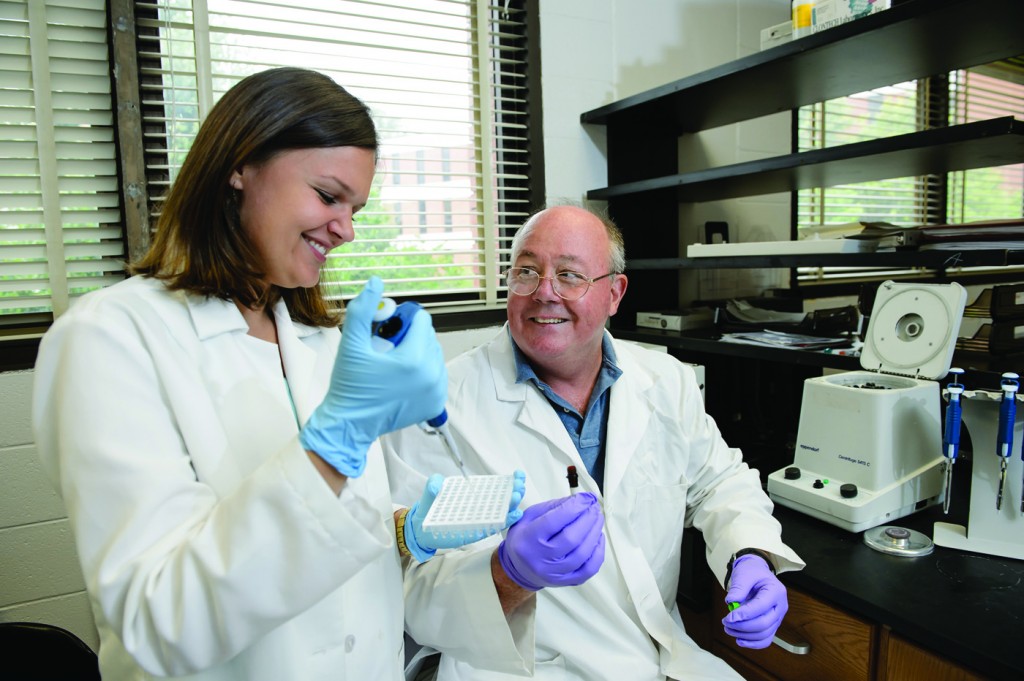
Or,
There’s more to indigo than blue.
If you’re grilling a steak, serve broccoli. That will help you avoid a problem with promiscuity.
Seriously. That’s the term scientists use for the AH receptor hanging out in your cells: promiscuous. It consorts with all kinds of shady characters, including the cancer-causing compounds that form when we’re charring a burger or steak on the grill. The AH receptor will bind to those compounds and, worst case, trigger runaway cell proliferation that leads us down the road to ruin. Compounds in broccoli, cabbage, cauliflower, and other cruciferous vegetables tend to block the receptor and turn the intruders away.
No, this won’t be another long lecture about eating your vegetables, worthy as that theme might be. This is a mystery story. A whodunit.
Most receptors are monogamous: They cleave to their usual partners, their dedicated ligands. They don’t sleep around. So why has nature afflicted us with this one promiscuous receptor and allowed it to lurk in the doorways of our cells, luring the villains inside? Villains that include not only the compounds in charred meat but toxic pollutants such as PCBs and dioxins, some of the deadliest toxins on Earth?
Scientists know that the AH receptor does have a legitimate role to play, especially in development, cell-cycle control, circadian rhythms, and general environmental sensing, but the ligands involved bind weakly and don’t hang around long enough to turn toxic. The receptor has been on the job for millions of years, since the very origins of vertebrate animals.
But we don’t know for sure how it got here, or what its natural partner—its endogenous ligand—might be. The AH receptor is, to date, an unexplained orphan of nature. That’s another word scientists use to describe it: orphan. Provenance uncertain. To muddy the waters, it also comes in one flavor for mammals but multiple flavors in lower vertebrates. Scientists don’t yet know why the receptor needs so many guises, but somewhere along the line, in its millions of years of evolution, many genes, including the AH receptor, saw the need to diversify, or duplicate. As vertebrates evolved these duplications were thrown out. For the purposes of our little story, we’ll talk about all the AH receptors as one.
Clues to our mystery of the renegade receptor have been turning up in the strangest of places. In the beards of pirates and the turbans of Tuareg nomads of the Sahara. In seashells. In a fish that eats poisonous slime. In thousands of years of Chinese herbal medicine. In a plant called indigo, a botanical powerhouse that changed the course of South Carolina history.
The AH receptor is an ancient puzzle with all of these pieces and more, and the puzzle has only recently begun yielding to science. Charles Rice and his students, concentrating on the immune system, have started to place a few pieces.
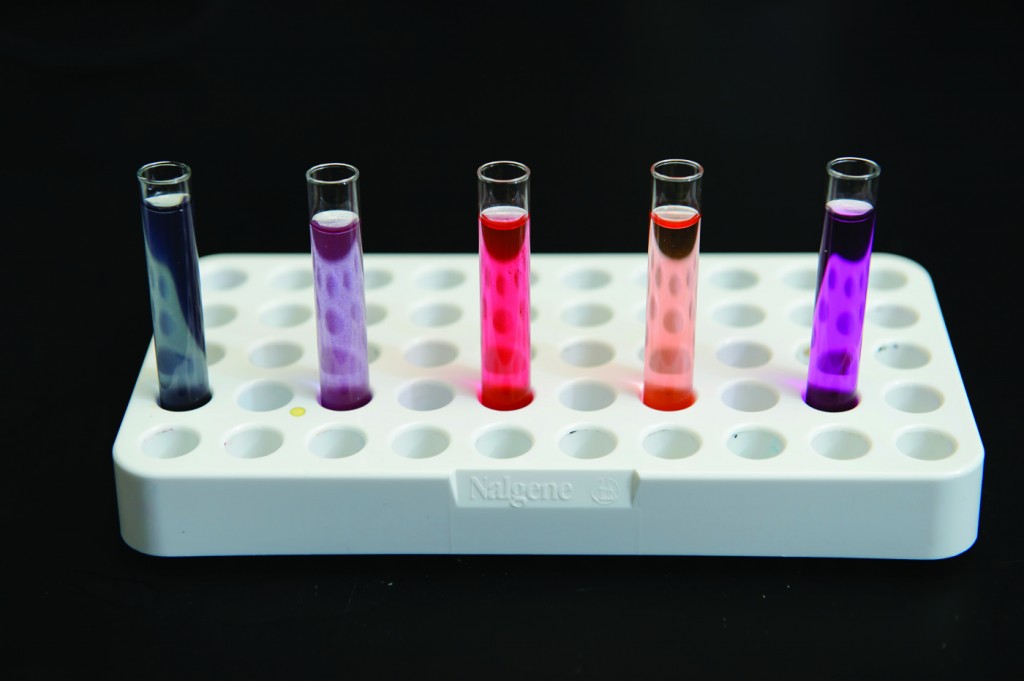
Photo by Ashley N. Jones.
Indigo: a red-state, blue-state kind of thing
Last fall, in this magazine, we ran a cover story about the history of indigo, a cash crop in colonial South Carolina that made fortunes here and abroad because African slaves could extract from its pulverized, decomposing tissues a vivid blue dye (see the colors of indigo). Rice read the story and emailed to say that we’d missed something. Indigo, he said, has an alter ego: indirubin, which is red.
“If you could go back to colonial South Carolina, and find a brick of indigo dye, somewhere inside that brick the color varies from blue to red,” Rice says. “Every time you put something around these molecules it changes the way they absorb or reflect light. It takes almost nothing to change that molecular structure, because they’re just different sides of the same thing.”
Two colors, two distinct sets of compounds. And the red version, Rice says, yields some potent magic of its own.
He knows this because he’s been studying red indigo for thirteen years, ever since a colleague invited him to a conference in Le Eyzies, France. The topic was indigo—the science and the history. That’s where Rice learned, for example, that Bluebeard the pirate used indigo to kill microbes that grew in his beard.
When Rice returned from that conference, coming home to a state whose flag shimmers indigo blue, he had a notion or two about the red side of indigo’s split personality, an isomer known as indirubin.
“The interesting thing about indigo and indirubin is that they’re both based on the natural product tryptophan, which is an essential amino acid we find everywhere,” Rice says. “So this process happens all around us in nature. If you look inside a seashell and see that dark blue with a reddish iridescence? That’s indirubin. And for hundreds and hundreds of years, people have dyed their turbans with indigo, and the most costly ones with the highest status have this iridescent sheen. That’s indirubin.”
The ancient powers of indigo and indirubin probably evolved as a self-defense strategy for plants and animals alike, Rice says. Bluebeard was onto something, when he colored his beard with the stuff. Certain mollusks produce blue and red indigo to guard against microbes, protecting eggs from infection. Other animals and plants produce it to shield against damage from ultraviolet light. So indigo, in each of its versions, seems to be one of nature’s oldest and best strategies for self-defense.
Humans don’t sport an iridescent sheen of self-protection, but we aren’t exactly strangers to indigo and indirubin. For one thing, our AH receptors readily bind to them both. For another, we can actually make the stuff, sometimes, in our bodies. “Under certain circumstances,” Rice says, “patients in a hospital can have too much tryptophan, and the bacteria in the gut can turn it into indirubin, and the patients pee red. It’s called purple urine bag syndrome.”
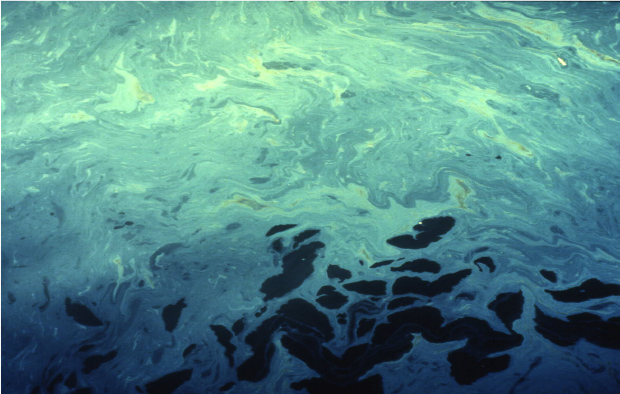
From China, a dose of detox tea
As he dug into the human dimensions of indigo’s history, Rice learned that people weren’t just wearing blue and red indigo to look better, they were also consuming it to feel better. “This goes back thousands of years, to Chinese herbal remedies,” Rice says. “The Chinese had this herbal tea, and it contained eleven different plant products, and it was used to help detoxify patients from whatever drug they were on, maybe even too much alcohol. It was also used to treat certain cancers and runaway fever. Eventually, they discovered that the active ingredient was this thing called indirubin.”
Indirubin, Rice learned, showed strong potential for reducing inflammation. Medically, that’s significant, because scientists have found that many human ailments, including heart disease, diabetes, and some cancers, can begin with inflammation.
But here, the plot thickens. While indirubin does fight inflammation, it also has a nasty habit of hooking up with—you guessed it—that promiscuous AH receptor. And when that happens, things can go awry in a hurry.
Why, scientists wondered, would indirubin, a natural compound as old as the hills, consort with the very same AH receptor as nefarious newcomers like PCBs and dioxins? Rice has some notions, but no final answer. He suspects that over millions of years of evolution organisms made good use of tryptophan-related or tryptophan-derived compounds, including indirubin, to stay alive, and may have done so via the AH receptor. If that’s the case, then indirubin may well be one of the natural, endogenous partners for the orphan receptor. And somehow the nasty carcinogens such as PCBs and dioxins usurped the receptor and its pathway, with deadly results.
Rice explains that most tryptophan-derived compounds that bind the AH receptor are metabolized and eliminated quickly, so the ill effects of AH activation tend to be minimal. PCBs and dioxins are difficult to metabolize, so the AH receptor is activated for a prolonged period, leading to severe toxicity.
Whatever its original function might have been, the AH receptor’s ancient affinity for hooking up with indirubin becomes problematic, if the goal is suppressing inflammation. Could that hookup be prevented?
Amy Anderson, a Ph.D. student in Rice’s lab, went to work on the problem, analyzing various indirubins, many of them derivatives concocted by medicinal chemists. To begin, she looked for indirubins that could fight inflammation. She found them.
At this point, we’ll need to introduce a rather warlike new character: the macrophage. Macrophages are soldier cells in our immune system; they defend against invaders. There are two kinds of macrophages, killers and rebuilders, known respectively as M1 and M2. The killers deploy and destroy, assailing enemy pathogens at the site of a wound—the short-term inflammation our bodies use to stop infection. The rebuilders, M2s, promote the proliferation of new cells to heal the wound. Trouble is, M2s also help proliferate tumor cells, and they show up in the kinds of long-term, chronic inflammation associated with heart disease and cancer. One goal, Rice says, is to “polarize” the macrophages to yield more M1s and fewer M2s.
So Anderson went looking for an indirubin that would not bind to the AH receptor and would fight the kinds of inflammation caused by M2s. Apparently, she found that too.
In her dissertation, which this spring earned her a Ph.D., Anderson reports that an indirubin known as E804 suppressed inflammation (she found very little M2 macrophage activity in the cell lines she tested) but did not seem to bind to the AH receptor, or at least not very well. When a compound does bind to the receptor, the body mobilizes a protein to detoxify the intruder. With E804, Anderson found no signs of the protein.
There are at least two possible reasons the protein didn’t show up, Rice and Anderson say. The first is that E804 did not bind to the receptor at all. That means, in theory, that E804, or something like it, might turn out to be safe for treating inflammation, assuming that researchers get similar results from studies with other cells lines and with laboratory animals. One possible application, Rice says, might be a topical ointment or cream for skin ailments such as eczema or psoriasis. Cosmetically, that would require some pharmaceutical finessing, because no matter how you formulate it, indirubin is red. “To take the redness out, you’d have to take the goodness out,” Rice says.
But a second possible explanation for why E804 didn’t mobilize the detoxifying protein is even more intriguing. What if E804 did bind to the receptor but did not cause toxicity? What if it’s a broccoli-like compound that sits on the AH receptor and blocks the door? What if it can shield our cells from toxins, even as it fights inflammation?
If that turns out to be the case, then the potential for medical treatments might well be huge. But all of this is highly speculative, at this stage. And the biologist in Rice keeps circling back to the heart of the mystery, the nature of this inscrutable, promiscuous orphan, the AH receptor. What is it up to, and why?
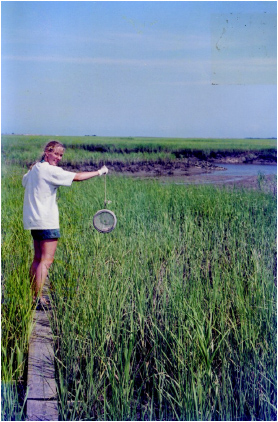
Enter the red herring
At this point in any respectable mystery story, you might expect a red herring. And sure enough, this is where the indirubin tale turns fishy. The fish at hand, in this case, is not a herring but something smaller: the killifish, a common sight around docks in brackish and marsh shallows of coastal waters.
Charles Rice grew up in Virginia, on the Chesapeake Bay, near Reedville, a fishing village famous for menhaden fisheries. For as long as he can remember, he’s been fascinated by the little fish that people call bull minnows, the Atlantic killifish. And for years now, he has studied a particular population of killifish unlike any other. They thrive in what Rice calls “one of the most polluted places on the planet.”
According to the Environmental Protection Agency (EPA), the Atlantic Wood Industries (AWI) superfund site includes forty-eight acres of land on the industrialized waterfront of Portsmouth, Virginia, and thirty to thirty-five acres of contaminated sediments in the southern branch of the Elizabeth River. From 1926 to 1992, AWI operated a wood-treating facility at the site, discharging into the surrounding waters creosote and pentachlorophenol (PCP), both of which attack multiple tissues and systems in the human body and are classified as probable carcinogens. For a time, the U.S. Navy leased part of the property from AWI and disposed of abrasives and sludge from the production of acetylene there.
The site’s toxic soup of creosote and various other chemical wastes is stubbornly persistent, Rice says. “Even today, you can jam a stick into the sediment, and these big blobs of oily stuff come up. People tell me that if you go out and walk in this place called Atlantic Wood, it will eat your rubber boots right off your feet.”
And yet, killifish in those waters are thriving.
“This population of killifish has adapted to the point where they’re absolutely tolerant of the contaminant,” Rice says. The fish live just as long as other killifish—about three years—and they grow even larger, perhaps because their natural predators steer clear of the pollution. “Things like blue crabs that feed on these killifish, they just scoot out as fast as they can,” Rice says.
Survival in such a toxic environment is no small feat, Rice says. “If you take fish from clean environments, fish that are not adapted to that site, and you expose them to sediments from the Atlantic Wood site, they get these typical-looking lesions, these sores that are breaking apart, and it’s really nasty.”
Now here’s the truly mystifying part. If you take the Atlantic Wood killifish out of their foul habitat and put them in clean water, they die. “They start forming those same kinds of lesions,” Rice says. “The histopathologist I work with, he just looks at it and says, ‘I can’t explain this. It’s bizarre.’”
To repeat: In clean water, the toxin-tolerant killifish die.
In a whodunit, this would be the ultimate plot twist. The victim was alive and well after having been poisoned with arsenic, stabbed with a knife, strangled with a rope, and bludgeoned with a wrench, but later dropped dead while being treated to a good rest, clean water, and wholesome food. Talk about the perfect crime.
This kind of thing was not supposed to happen. But it does.
“As an immunologist,” Rice says, “my question has always been, if the fish live in this toxic environment, surely their immune system is blown out. It has to be.” He shakes his head no: “They’re basically healthy enough to reproduce, as long as they stay put.”
The Atlantic Wood killifish swim, eat, grow, and reproduce as normal. But that’s not to say they swim around unscathed. Killifish from the site show high levels of chronic inflammation, and they don’t possess the normal blood-circulating antibodies that other fish depend upon for defense against bacteria. And their livers? A disaster zone, Rice says. “By the time these fish become adults, between seventy and eighty percent of them have liver cancer, and almost all of them have some kind of liver damage, which you’d expect in that environment. Almost their entire liver can be a tumor, and they’re still looking fine from the outside. If that happened to us, we’d be dead.”
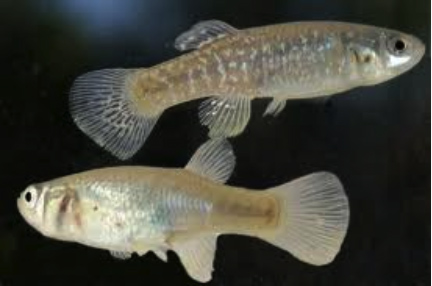
What’s not killing the killifish?
For scientists studying cancer, these improbably adapted killifish represent a living laboratory of chemical carcinogenesis. But how can any killifish survive without a full of set of antibodies and a functioning liver? And what kills them, when they take a long swim in clean water?
Rice has suspicions, but no smoking gun. At least not yet.
He has been looking for clues at the site itself, the shallow waters around Atlantic Wood. “What is unique about this spot is that when the tide is low you see a thin biofilm of algae, bacteria and stuff, growing on top of the sediments. You don’t see that anywhere else.”
When you take a living killifish out of the water, he explains, it throws up whatever it’s been eating. In clean-water habitats, that typically would include a lot of baby crabs and small worms. But the Atlantic Wood killifish disgorge almost exclusively a black-and-green slime.
“The thing is, I haven’t seen this biofilm anyplace else,” Rice says. “One of my colleagues at the Virginia Institute of Marine Science thinks that this biofilm is completely adapted to that environment, and lives on those contaminants there as a food source.”
The killifish are eating the biofilm, Rice thinks, and he and his students have explored the possibility that the biofilm itself somehow helps protect the fish. But an initial round of experiments with cell cultures did not find evidence to support that idea, so the mystery remains. But it seems likely, Rice says, that the AH receptor holds the key. His colleagues at Duke University and Woods Hole Oceanographic Institution have found that modifying the AH receptor prevents some of the deformities that appear after fish larvae or embryos adapted to clean water are exposed to toxins. “So we know that the AH receptor is involved, somehow,” Rice says. “We don’t know exactly how, yet, but it’s involved in adult fish.”
The biology of all this remains rather murky and rather noir, much to Rice’s fascination and delight. For one thing, the adaptation of killifish to a toxic habitat may not result from genetic mutations of the kind we usually think about when we’re talking evolution. The changes may instead be epigenetic, Rice says, which means that a code of biochemical tags called methyl groups may be turning certain genes on or off. Flip the switches one way, the fish can gobble black slime and thrive in toxic water. Flip the switches another way, they die.
Whatever flips the switches, the renegade receptor is no doubt involved. And to learn that receptor’s true calling, its reason for being, is one of those quests that drive a scientist like Charles Rice to spend a good part of his summer collecting black slime from toxic sediments and hauling it back to the lab like so much Teenage Mutant Ninja Turtle ooze. What he learns about the stuff, well, that will have to play out in the chapters to come.
Charles D. Rice is a professor of biological sciences in the College of Agriculture, Forestry, and Life Sciences. Funding from the National Institutes of Health has supported the research described here.