Good Vibrations
by Neil Caudle
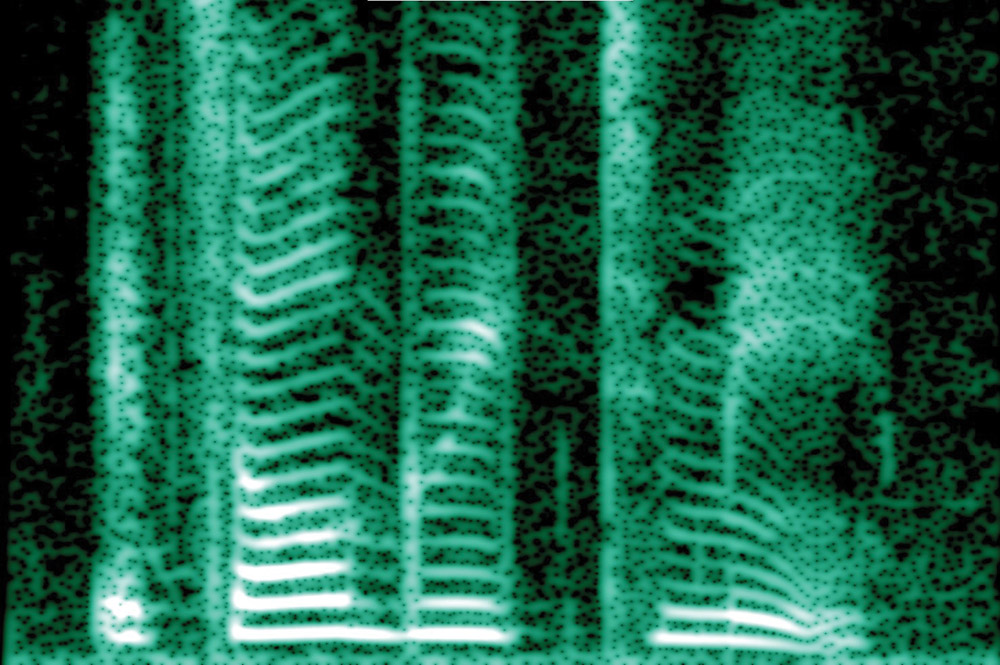
A spectrogram (0-5000 Hz) of the sentence “it’s all Greek to me” spoken by a female voice. In this case, the pattern is serene. Image: Mysid Dvortygirl, Creative Commons.
When Janis Joplin, rock’s legendary queen of self-destruction, belted out her raspy blues, she was destroying her pipes. Her vocal cords pounded each other so hard that they couldn’t recover before the next all-out assault. Slowly, they stiffened with scar tissue, slowly turning rigid and silent as stone.
But for most of us, vocal cords are remarkably resilient. We can scream ourselves hoarse at a football game and, if we don’t overdo it, we’ll be fine in just a few days. In fact, the resilience of our vocal cords is a marvel of physiology so extraordinary that it hints at the nature of healing. Ken Webb has been finding those clues in what he calls the matrix.
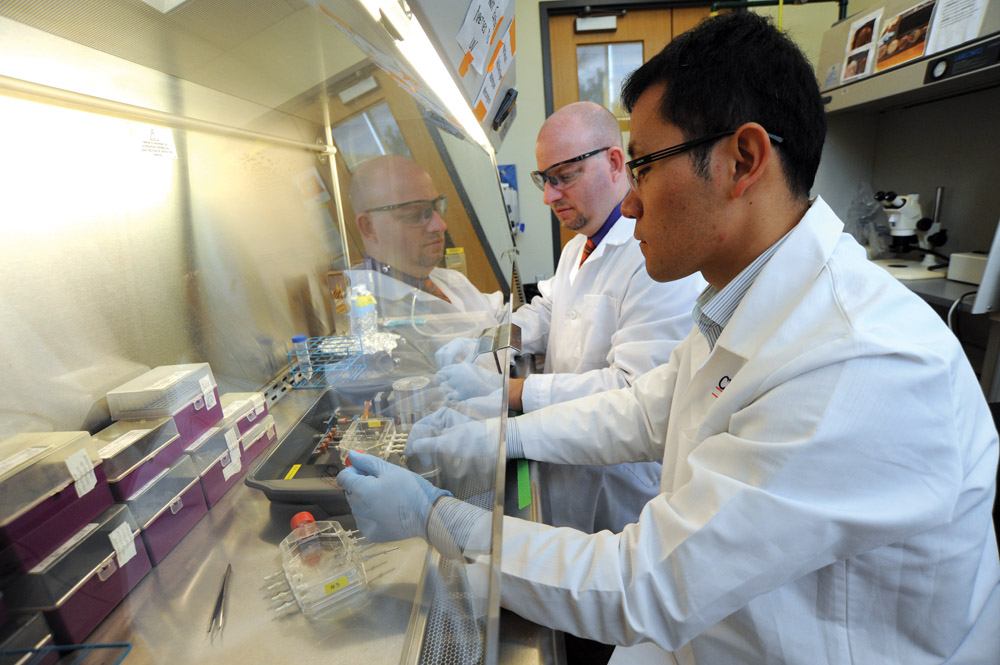
Finding the vibe: Ken Webb (left) and Sooneon Bae, his Ph.D. student, have found evidence that vibration, coupled with the strain of cyclic tension, helps vocal cords remain flexible and avoid scarring. Using a bioreactor Bae built (below), the team tests vocal-fold tissue in various combinations of tension, vibration, and relaxation. Their results suggest the potential for using vibration in treatments for fibrotic disease.
Locked in the matrix
The extracellular matrix is a mesh-like structure that holds tissues and organs together. It is made mostly of collagen, which forms tough, skinny strands. When we are injured, collagen erects a quick scaffold to rebuild the matrix and help tissues heal. Sometimes, we overbuild the scaffold, and that leads to scarring.
“A hallmark of severe scarring is an excess accumulation of collagen,” Webb says.
Until we abuse them, our vocal folds, as scientists prefer to call them, don’t require much collagen to hold them intact. To vibrate freely, they need flexibility, not rigid strength.
Unlike other tissues, a healthy set of vocal folds is resilient enough to recover from constant wear and tear. A Beach Boys tune? No problem. But when we Joplin our voices, we traumatize the tender folds. If the damage is severe, or if we don’t give the folds enough rest to recover, collagen accumulates and the scarring begins. It’s the body’s way of saying, “If you’re going to play that rough, I’ll put on a suit of armor.”
The result, Webb says, is vocal pathology—the kind of damage that afflicted not just rockers like Joplin but, at the other end of the Zeitgeist, Julie Andrews.
Built for action
Vibration alone is not really the problem. In fact, our vocal folds grow and thrive with the right kind of vibe.
“I think there’s a lot of evidence to support the idea that mechanical stimulation promotes tissue-specific matrix accumulation,” Webb says. “So in tissue engineering, people use bioreactors to develop the matrix and therefore the appropriate functional mechanics.”
Engineering a heart valve, for instance, can benefit from pulsation in a bioreactor, to simulate normal, rhythmic flow.
If our tissues are healthy, the matrix can recover from repetitive wear and tear. We can sing a cantata or go for a jog without kicking collagen into overdrive. But in fibrotic disease, something upsets the equilibrium, and collagen accumulates. Webb and his students have been learning what that something is. And their findings have several interesting implications for medical science.
Consider diseases such as pulmonary fibrosis and cardiac fibrosis, both of which are painful and deadly. Renal fibrosis, which attacks the kidney, is especially common in late-stage diabetes, an increasing threat around the world.
The culprit in each of these diseases is an overabundance of collagen, which forms a great many very tough fibers. Scientists think that each disease originates from some form of minor but persistent injury that stimulates an unusually high concentration of growth factors, molecules associated with matrix production.
How are the vocal folds, whose vocation is vibration, able to repair the minor wear and tear resulting from normal speech without developing fibrotic disease? If a vocal fold can protect itself from fibrosis-inducing molecules, why can’t a lung or a kidney or a heart?
The answer, oversimplified, is that the phenotypes are different. Our phenotypes—the versions of our physical selves that can actually be observed—reveal themselves when some molecule flips a switch that says, for example, “You will have a healthy lung,” or “You will have a fibrotic lung.” We store in our genes an instruction set for these and many other phenotypes, with switches to control the selections.
So Webb and his students went looking for the switch.
“Our original direction was to try to use molecular biology to identify a molecular switch, activated by vibration, that would ‘turn off’ the pathways leading to fibrotic disease,” he says, “and then perhaps to design a drug to activate that switch in patients with fibrotic disorders.”
In search of a switch
To search for this switch, Webb and Sooneon Bae, his Ph.D. student, took a close look at vocal cords, trying to understand them mechanically as well as biologically. They used a bioreactor to apply mechanical stimulation to living cells, simulating the cycle of tension and relaxation as well as the high-frequency vibration that occurs in our vocal cords.
“Normally when we speak the tissue is tensed or stretched,” Webb explains. “It vibrates under tension, and then it relaxes. This is the oscillatory pattern of our speech.”
So the researchers built the bioreactor to simulate both the tension and the vibration. One side of the device creates the strain and another uses an electromagnetic speaker coil that vibrates the samples at 100 hertz, in the lower end of the range for human voices.
As Webb and Bae simulated the mechanics, they also studied the biology of what happens to cells under tension and vibration. Webb’s collaborator at the Medical University of South Carolina (MUSC), Jeremy Barth, used microarray analysis—a high-tech tool for analyzing the expression levels of all messenger RNAs expressed by the cells. Because messenger RNA molecules carry genetic information from the DNA, the researchers could track how the cells responded to the cycle of strain and vibration.
Getting off the dime in the new paradigm
Ken Webb has a message for students—and for the rest of us, too: Times have changed. The paradigm has shifted.
A generation or two ago, Webb says, success usually meant following the blueprint for a good life: Go to college, get a good education, find a good job, and work your way up.
“That’s just not as applicable today,” Webb says. “You can’t count on that job being there, just because you’ve finished college. Fields like bioengineering are too competitive. Students today, even when they are sophomores, really need to view themselves as entrepreneurs.”
That means learning how to launch one’s career as an enterprise, with risks and potential rewards, Webb says. “The students who will be the most successful are the ones who realize they will have to build their own skill sets, build their own résumés, and market themselves.”
In fields evolving as rapidly as bioengineering, he says, a good way to stay relevant, add skills, and begin to build an enterprise is to do hands-on research and engineering as part of a team, creating new knowledge and products. Webb points, for example, to the senior design program in bioengineering, which has yielded patented inventions, start-up companies, and national awards—an auspicious beginning for any group of aspiring entrepreneurs.
If you ask him about his own research, Webb will gladly walk you through it, and it’s clear that he finds it exciting—both the quest and the potential for beneficial applications. But in the end, that’s only half the story.
“The best part of the work is mentoring students,” he says. “There’s nothing more satisfying than seeing a student grow and develop and go out and become successful.”
Telltale molecules
The experiments uncovered several clues to what happens in fibrotic disease. For one thing, the team identified a number of cytokines—molecules involved in cell regulation, signaling, and growth—associated with fibrosis.
“When a patient has pulmonary fibrosis, renal fibrosis, or cardiac fibrosis, there are certain molecules that are usually present in higher concentrations,” Webb says. “Interestingly, vibration activates those molecules strongly.”
But if vibration in the normal range for vocal cords triggers the signals implicated in fibrosis, why does it take a Joplin-like assault to begin forming scars?
Somehow, healthy vocal cords avoid fibrosis.
“Even at later time points the collagen does not become activated,” Webb says. “It seems that vibration initially causes something of a pro-fibrotic response that would be detrimental, but at the same time it’s somehow inhibiting the normal consequence of these molecules, which would be excess collagen accumulation.”
In other words, vibration in the vocal folds fires up trouble but quickly snuffs it out. How and why? The answer, Webb suspects, is that somehow vibration is also inhibiting the normal signaling of these molecules.
His experiments reveal numerous alterations in the signaling pathway for a molecule called transforming growth factor beta 1 (TGF beta 1), a molecule that tends to induce its own negative feedback, Webb says. TGF beta 1 may, in the case of vibration under tension, be self-defeating.
“But that’s getting pretty speculative,” Webb says.
Negative feedback
Webb and Bae conducted an experiment in which they cultured collagen-producing cells under cyclic strain but with no vibration. The result was lots of collagen. “A week later, you can actually macroscopically test these samples,” Webb says, “and they’re stiffer.”
But when Webb’s team combined strain with vibration, the results were very different. There was no stiffening, no apparent excess of collagen. Vibration seems to override the fibrotic signaling normally activated by cyclic strain.
To apply this concept to a treatment for fibrosis, Webb needs to know exactly how this works, at the level of molecules and cells. “We have a couple of hypotheses,” he says.
In normal wound healing, the cells responsible for a lot of collagen production and scarring are called myofibroblasts. In fibrotic disease, these cells tend to gang up in great numbers and hang around too long. But during normal healing, the myofibroblasts chill out, after a while. They ease into what Webb calls senescence, becoming inactive; some of them die.
Other research groups have studied this senescence, and from their results, Webb sees evidence that vibration influences a set of genes that have been linked to the senescent phenotype. “The cells start to secrete certain molecules that affect themselves and their neighbors to down-regulate this matrix-accumulation phase of the wound healing response and turn it off,” he says.
Could that happen in other parts of the body? In a fibrotic lung or heart or kidney, for instance?
“We believe that it could be useful in other places,” Webb says. “To give you one example, ultrasound is used in bone healing, as an aid to fracture healing. And that ultrasound device may induce some degree of vibration. To my knowledge, the mechanism is not really understood. It’s just empirically known that it works.”
Webb thinks it is possible that high-intensity, focused ultrasound might provide another approach by delivering vibration and its anti-fibrotic benefits directly to diseased tissues. This approach might even turn out to be more effective than the drug he originally had in mind. He’s been talking with colleagues about how to pursue the new angle. Meanwhile, he still needs to figure out, at the level of proteins and molecules, exactly what happens to keep a healthy vocal fold in shape.
For the time being, we’ll just credit those good, good, good—good vibrations.
Ken Webb is an associate professor and associate chair of undergraduate affairs in the Department of Bioengineering, College of Engineering and Science. Image of Ken Webb and Sooneon Bae by Patrick Wright.